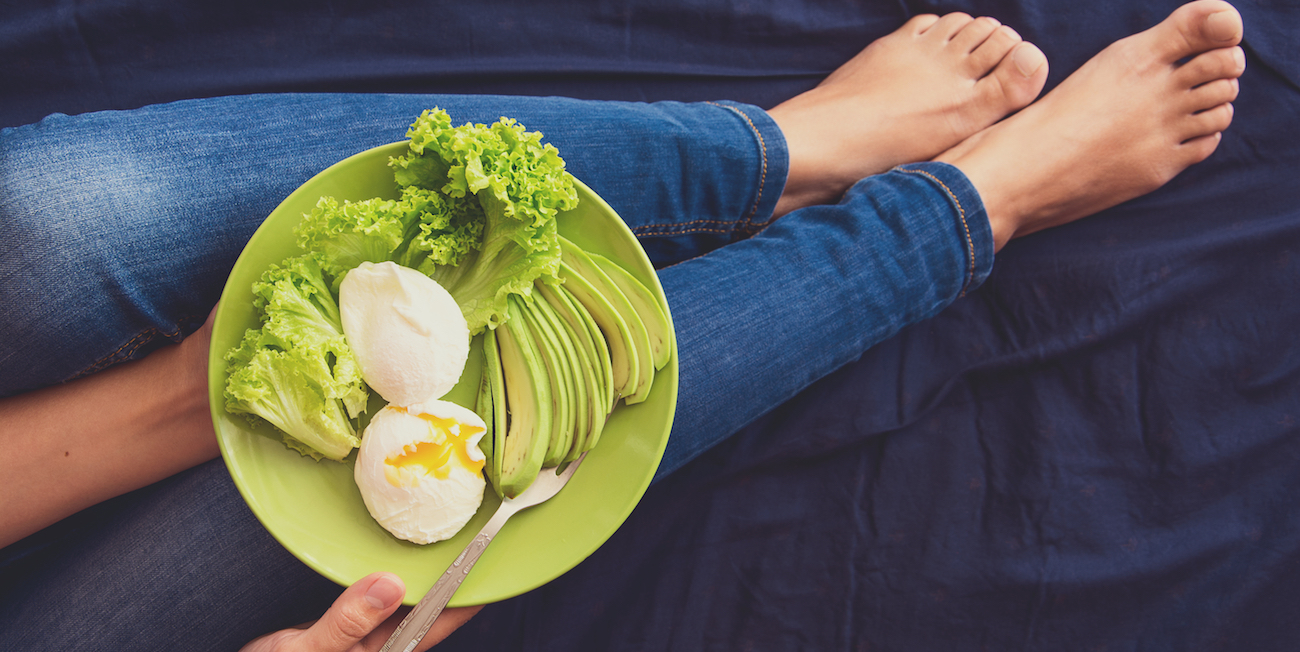
Why Humans Don’t Need Dietary Carbohydrates to Thrive

Snap Shot
When discussing carbohydrate restriction, two fallacious arguments related to the energy needs of the brain and the sustainability of a ketogenic diet are often levied against the use of a well-formulated ketogenic diet in practical therapeutic medicine:
- The human brain burns 600 kcal per day, and this translates to a 150 gram per day glucose requirement to meet its energy needs, and
- No one can follow a ketogenic diet long term.
In the peer-reviewed medical literature over the last 5 decades, these arguments against the safety and sustainability of nutritional ketosis have been proven false over and over again, most recently with the 2-year results of our Indiana University Health study¹.
We have addressed the necessary components of a well-formulated ketogenic diet that most people can follow for years if properly informed and supported. The specific topic that we want to address here is how both the brain and body can function as well—or even better—on a diet with little or no dietary carbohydrate compared to the typically promoted low fat, high carbohydrate ‘healthy diet.’
Published science has shown that ketones that are produced from either dietary fats or triglycerides stored in our adipose tissue reserves are an excellent fuel for the brain. Further, we now know that these ketones produced by the liver also have multiple beneficial effects on the heart, kidneys, and other organs that appear to translate into improved longevity²,³,⁴. Additionally, new research has highlighted that skeletal muscles, even those of competitive athletes, are not solely dependent on high dietary carbohydrate intake for glycogen replenishment and performance⁵.
However, up until 5 years ago, however, we struggled to understand the mechanism(s) of these additional beneficial effects. Now we know why this long-discounted physiology can play a dominant role in our health and well-being. In addition to the fact that ketones are a cleaner-burning fuel (i.e., producing fewer free-radicals) than glucose when used by the brain and other organs, the primary ketone beta-hydroxybutyrate can also function as a signal to activate genes that regulate our defenses against oxidative stress and inflammation³.
How the body shifts its primary energy source from carbohydrates to fats and ketones is anything but simple. This process, which we have named ‘keto-adaptation,’ starts within days but takes a considerable period of time to fully develop. And even after it is complete, the result is not an absolute exclusion of glucose from the body’s fuel supply. Rather, the need and use of glucose is dramatically reduced, while at the same time pathways that salvage products of partially metabolized glucose (e.g., pyruvate and lactate) for recycling into fuel and other beneficial metabolic intermediates become more finely tuned. The result is maintenance of normal blood glucose and muscle glycogen levels that can be sustained without the need for dietary carbohydrate intake.
Physiologic Role of Carbohydrates
The belief that the brain and central nervous system need carbohydrates to function properly is often supported with the circular logic that the brain uses glucose therefore it needs glucose, and it needs it because it uses it. The hole in this argument is that the brain does not in fact need glucose. It actually functions quite well on ketones. Stating it another way, the presumed requirement for glucose by the brain is a conditional need that is based on the fuel sources dictated by one’s choice of diet. A ketone-suppressing diet (i.e., any diet supplying >30% of energy from the combined intakes of carbohydrate and protein) essentially forces the brain to rely on glucose for fuel.
It is true that some cells within the body do require glucose. For example, red blood cells, parts of the kidney, and the epithelial cells that cover the lens of the eye are primarily glycolytic because they lack mitochondria and thus are dependent upon glucose to function. This is also partially true for the fast-twitch muscle fibers (which have fewer mitochondria than slow-twitch muscle) used for high intensity exercise like weight lifting and sprinting. But in all of these cases where glucose is broken down to lactate, the body then has a choice—cells with mitochondria can further oxidize the lactate to CO2 and water, or the body can recycle that lactate back to glucose.
Evidence That the Brain Can Function on Ketones
The simplest experiment that demonstrates the brain’s ability to function on ketones is the observation that humans can tolerate total fasting with normal mental function for durations of 30-60 days. Interestingly, during prolonged starvation, muscle mass and other important structures in the body progressively lose mass and function. The brain, however, is fully protected against the starvation catabolism that depletes the rest of the body. Elegantly done studies that measured glucose and ketone levels in arterial blood going into the brain compared to these fuels in the jugular vein coming out of the brain, indicated that ketones are in fact able to supply the great majority of the brain’s energy. But because even prolonged starvation does not reduce the blood glucose level below the ‘low normal’ range, these observations did not prove there is not a small but significant glucose requirement for the keto-adapted brain.
This question was addressed directly many decades ago when two prominent research groups undertook similar experiments to evaluate mental function in starvation-adapted patients whose blood glucose was reduced to very low levels by an infusion of insulin⁶,⁷.
[Although we are sharing this as valuable published information, we also need to note that while these experiments were considered acceptable in the day, they would not pass ethical muster today due to the risks for the patients compared to the benefits of the accrued knowledge.]
Both studies involved severely obese patients who had been on total fasts under continuous inpatient observation for from 30 to 60 days. In the study by Drenick et al., 9 participants with blood BOHB (beta hydroxybutyrate) in the 7–8 mM range were administered a single bolus of insulin sufficient to transiently drive blood glucose values down to a mean of 36 mg/dl (with some patients’ values going as low as 9 mg/dl). Despite causing profound hypoglycemia to levels normally associated with coma or death, none of these patients experienced any symptoms associated with hypoglycemia. Moreover, measures of urinary catecholamines that are indicative of the body’s counter-regulatory stress response to hypoglycemia were not elevated, despite these brief but profoundly low blood glucose values.
In the other study reported by Cahill and Aoki⁷, 3 obese males adapted to prolonged fasting were administered insulin via a slow constant infusion over 24 hours. In this case, blood glucose levels declined gradually, but eventually reached a mean value of 25 mg/dl, while blood BOHB stayed in the 4–6 mM range. With this method of insulin administration, blood glucose values under 36 mg/dl were sustained for 10–12 hours, but again the patients exhibited no clinical signs of hypoglycemia or a counter-regulatory hormonal response.
What these two dramatic (but risky) studies demonstrated is clear evidence of normal brain function in the virtual absence of glucose when sufficient ketones are available. This offers us the unique perspective that when consuming a carbohydrate-rich diet, the predominate source of fuel for the brain is glucose; not because it is needed but because the other natural and highly effective brain energy source has been shut off. But under conditions of consistent nutritional ketosis, the brain adapts to the presence of ketones by enhancing their uptake and oxidation, thus protecting cognitive and CNS function⁶.
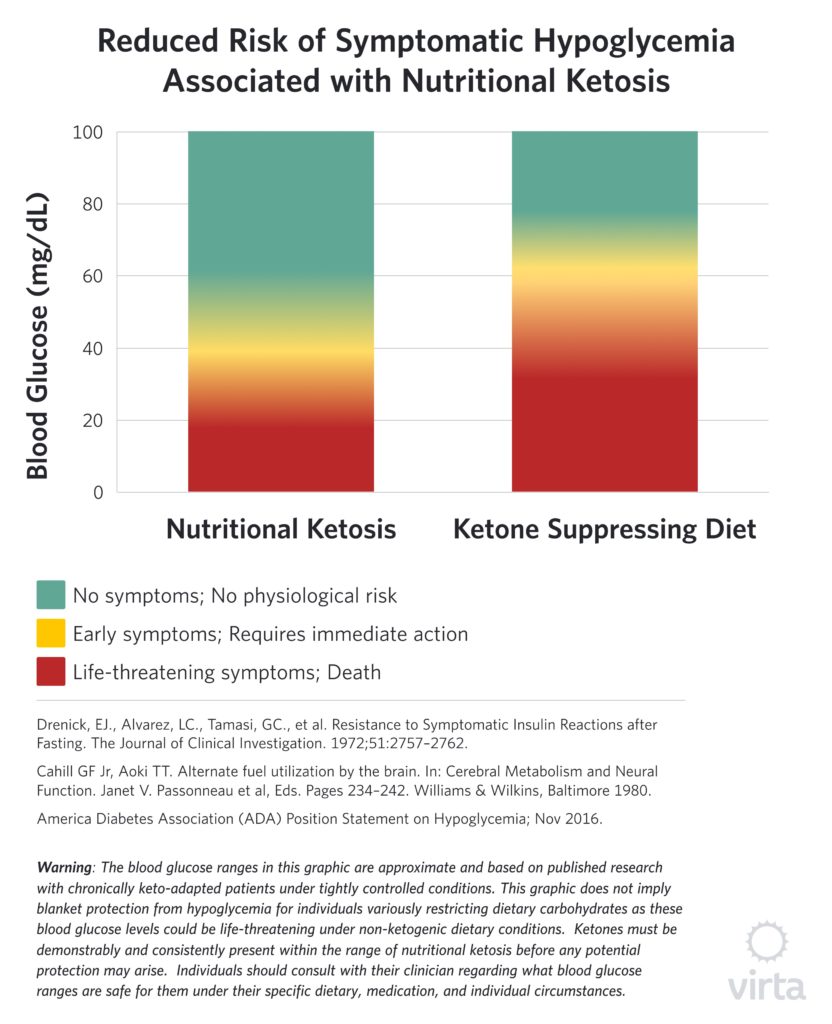
It should be noted that these studies showing potent neuro-protection by ketones under conditions of profound hypoglycemia involved small groups of patients with blood ketones in the 4–8 mM range, whereas nutritional ketosis values tend to be lower—i.e., in the 1–4 mM range. We do not have the results of similar human studies with purposefully-induced hypoglycemia, and modern ethical standards appropriately prevent such research. However, in managing numerous patients with type 2 diabetes taking hypoglycemic medication, we have observed many instances of moderate hypoglycemia without the expected symptoms when blood BOHB values are in the nutritional ketosis range. Also of note, is the fact that the brain favors ketones over glucose as indicated by preferential uptake of ketones even when glucose is elevated⁸. This also appears to be the case in the heart.
Essentials of Keto-Adaptation—Glucose Conservation and Salvage
It is important to remember that just because one doesn’t consume dietary carbohydrate does not mean the body is completely lacking in glucose. Whether on a total fast for weeks⁶,⁷ or following a meat-and-fat-only ketogenic diet for a month⁹,¹⁰, blood glucose values remain in the normal range both at rest and during exercise. This occurs because the body is quite capable of synthesizing all of the glucose it needs from various gluconeogenic precursors, while at the same time strictly limiting its rate of carbohydrate oxidation.There are at least five sources of these glucose precursors:
- breakdown of muscle to supply amino acids for gluconeogenesis;
- breakdown of dietary protein to supply amino acids for gluconeogenesis,
- glycerol released from the hydrolysis of adipose tissue triglyceride or dietary triglyceride;
- recycling lactate and pyruvate from glycolysis; and
- acetone produced by the spontaneous breakdown of acetoacetate to acetone that can be used for gluconeogenesis.
This last source is a bit surprising, as it is actually a small but significant pathway for the production of glucose from fatty acids¹¹. The conditions for and the amounts provided by these various sources of gluconeogenesis are shown in the following table.
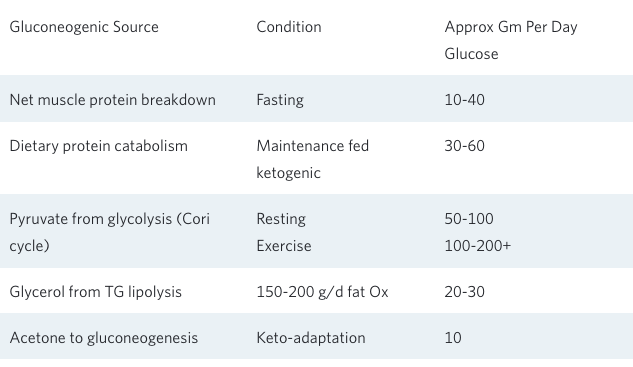
What this table clearly demonstrates is that whether during a total fast or a ketogenic diet without carbohydrate containing foods, new or recycled gluconeogenic substrates provide for the generation of anywhere from 100–200 g/d of glucose. Add to this up to 50 g/d of dietary carbohydrate as part of a well-formulated ketogenic diet, and it becomes clear why nutritional ketosis is well tolerated under a variety of challenging conditions.
The obligate other half of this balancing equation is the body’s ability to strictly limit its net use of glucose as an oxidative fuel. The extent of this conservation can be appreciated from indirect calorimetry data from keto-adapted adults at rest and during endurance exercise. In both untrained and highly trained individuals, this indicator of total body fuel use shows that approximately 90% of the body’s energy is being supplied by fat or ketones derived from fat⁵,⁹,¹⁰.
Lessons from Low Carbohydrate Athletes
Perhaps the situation perceived as most challenging for someone on a ketogenic diet is the ability to maintain glucose/glycogen reserves with prolonged, high intensity exercise. For a greater part of the last century, the accepted paradigm has been that one’s initial muscle glycogen is positively correlated with the ability to sustain endurance performance during moderate-to-high intensity exercise¹²,¹³. However, given that even with ‘optimized’ muscle glycogen obtained by using a carbohydrate-loading diet strategy, an endurance athlete has a peak total body glycogen content of only about 2000 kcal. To attempt to simultaneously train the muscles to use more fat and reduce one’s dependency upon glycogen in order to extend performance creates somewhat of a metabolic oxymoron. This is because very high insulin levels induced by carbohydrate loading actually suppress adipose fatty acid release and oxidation.
To explore this further and assess the limits of human fat oxidation during exercise, a research team from the Netherlands studied 300 adults, examining their maximum fat oxidation during exercise¹⁴. They reported the maximum rate of fat oxidation for the best individual fat burner in this group (which included a number of highly trained athletes) to be 0.99 grams of fat per minute. However long before that, one of us reported that bicycle racers who were keto-adapted for just 4 weeks were able to burn fat at 1.5 grams of fat per minute¹⁰. Based upon the before and after muscle biopsies taken in this study, after keto-adaptation these bike racers were able to perform the same amount of work while using just one quarter the amount of muscle glycogen. This was the first study that clearly disconnected muscle glycogen from endurance performance in keto-adapted athletes.
However, the best demonstration of this disconnect was recently published by Jeff Volek’s group⁵. We recruited 20 competitive ultra-runners, 10 of whom followed a traditional high carbohydrate diet and the other 10 had been following a ketogenic diet for at least 6 months (mean diet duration 22 months). The ketogenic diet group reported an average daily carbohydrate intake of 64 grams and had a mean fasting serum BOHB of 0.6 mM.
After baseline testing, these runners were asked to do a 3-hour run at race-pace on a treadmill—essentially an indoor marathon. Surprisingly, both groups had similar muscle glycogen levels before the run, and they also both mobilized similar amounts (about 80%) of their glycogen during 3 hours on the treadmill. But indirect calorimetry testing (measuring 02 consumption and CO2 production) indicated that almost 90% of ketogenic runner’s net energy use was from fat. This result is a clear indication that glycogen mobilization does not equate with carbohydrate oxidation in the keto-adapted state. Rather glycogen stores can be optimized and available for anaerobic (aka glycolytic) muscle function and then quantitatively recycled back to glucose by the liver. An even more astonishing example of being able to maintain normal muscle glycogen while consuming very little carbohydrate during repeated days of exhaustive exercise has been reported in trained sled dogs¹⁵,¹⁶.
Why Some Experts Still Claim that We Need Dietary Carbohydrates
In addition to the commonly stated but flawed arguments for dietary carbohydrate that we have addressed above—i.e., that the brain and some other tissues are obligate carbohydrate burners and that carbohydrates are required for exercise—there are a number of other reasons often used to support the idea that we need to consume carbohydrates above levels that facilitate nutritional ketosis.
The liquid protein diet debacle. With the publication of the book ‘The Last Chance Diet’ in 1976, a profoundly flawed diet with overt electrolyte and mineral inadequacies was promoted to the public, resulting in over 60 cases of sudden death reported to the CDC in the following few years. Rather than actually identifying the true underlying cause, expert opinion was that ketones were toxic to the heart¹⁷,¹⁸. Despite our publishing multiple rigorous studies demonstrating excellent maintenance of heart rhythm and function when adequate electrolytes and minerals are supplied during nutritional ketosis⁵,⁹,¹⁰,¹⁹ this flawed conclusion remains commonly held by many medical practitioners and scientists to this day. That notwithstanding, there is absolutely no scientific basis for the claim that dietary carbohydrates are necessary to prevent the buildup of damaging levels of ketones (AKA ‘toxic byproducts of fat metabolism’).
The myth of adrenal fatigue. In both general clinical experience and in some published research, poorly formulated low carbohydrate diets are understood to cause headache, fatigue, exercise intolerance (aka ‘keto flu), and adrenergic depletion (20). This study by DeHaven—The Yale Turkey Study—has been discussed in our prior blog post. In brief, they administered a protein-only diet to obese women for 4–6 weeks containing severely restricted amounts of sodium and potassium. The resulting impaired protein metabolism and profound hypotension was due to overt electrolyte inadequacies, not nutritional ketosis as the authors claim. These and other findings presented where subjects did not receive adequate electrolyte replacements have been used to paint a picture of physiological stress that can be brought on by a ketogenic diet, despite numerous studies indicating no increased catecholamine response in keto-adapted subjects⁶,⁷.
Thyroid dysfunction secondary to nutritional ketosis. In the context of the common observation of impaired energy and exercise tolerance when nutritional ketosis is combined with inadequate intakes of electrolytes, it is tempting to blame this on impaired thyroid function. However, this common conclusion does not stand up to basic scientific scrutiny. Yes, the blood level of the active thyroid hormone T3 typically falls by 30–40% in the first few weeks of a well-formulated ketogenic diet, but this is not accompanied by any signs or symptoms of clinical hypothyroidism. As discussed in our prior blog post Does Your Thyroid Need Dietary Carbohydrates?, this change is due to a marked reduction in thyroid hormone resistance (similar to the concurrent improved insulin resistance) during nutritional ketosis. Therefore, this is a healthy response and not a sign of endocrine dysfunction.
Sleep patterns are disturbed by a ketogenic diet. Many people report that they sleep less when in nutritional ketosis. We have recently addressed this question in a study of our patients in the Indiana University Health study. We found that global sleep quality, sleep disturbances, and daytime dysfunction parameters all were significantly improved. In addition, the proportion of patients reporting poor sleep was significantly reduced after 1 year²¹. A partial explanation for the mechanism of these benefits may be that the brain’s breathing response to CO2 buildup is improved during nutritional ketosis²².
We need more dietary fiber than is possible on a ketogenic diet. In addition to promoting colon health, there is now strong evidence that short-chain fatty acids (SCFAs) produced from colonic fermentation of fiber also improves brain health. And it is indeed true that the combination of a very high fiber intake plus adequate carbohydrate restriction to sustain nutritional ketosis is difficult to achieve without the use of purified fiber supplements. But what we point out in our blog post on fiber is that the production of beta-hydroxybutyrate can provide many-fold more SCFAs to the brain than a very high fiber diet combined with an optimized microbiome. Thus, the moderate level of fiber that one can achieve with a real-food well-formulated ketogenic diet should be more than adequate to maintain organ health throughout the body.
Conclusions
The need for dietary carbohydrates is often a topic of misunderstanding and misinformation. Although some specific tissues in the body do have certain glucose requirements, these requirements are easily met by gluconeogenic sources within the body without the need for dietary carbohydrate intake. There are also some people who claim a behavioral ‘need’ for bread, but that soon passes after a few weeks of keto-adaptation. The fatigue, stress, impaired cognition and reduced performance that are often used to argue for the need for carbohydrate are more aptly attributable to improper implementation of a well-formulated ketogenic diet, inadequate electrolyte replacement, and/or insufficient time for keto-adaptation. When used correctly, a ketogenic diet can be a safe and sustainable therapeutic tool as well as a means to help promote wellness and performance.
The information we provide at virtahealth.com and blog.virtahealth.com is not medical advice, nor is it intended to replace a consultation with a medical professional. Please inform your physician of any changes you make to your diet or lifestyle and discuss these changes with them. If you have questions or concerns about any medical conditions you may have, please contact your physician.
This blog is intended for informational purposes only and is not meant to be a substitute for professional medical advice, diagnosis, or treatment. Always seek the advice of your physician or other qualified health provider with any questions you may have regarding a medical condition or any advice relating to your health. View full disclaimer
Are you living with type 2 diabetes, prediabetes, or unwanted weight?
- Athinarayanan, SJ., Adams, RN., Hallberg, SJ., et al. Long-Term Effects of a Novel Continuous Remote Care Intervention Including Nutritional Ketosis for the Management of Type 2 Diabetes: A 2-year Non-randomized Clinical Trial. bioRxiv 476275
- Crawford, PA., Crowley, JR., Sambandam, N., et al. Regulation of myocardial ketone body metabolism by the gut microbiota during nutrient deprivation. PNAS. 2009;106(27):11276-11281
- Newmam, JC., Verdin, E. β-hydroxybutyrate: Much more than a metabolite. Diabetes Res Clin Pract. 2014;106(2): 173–18
- Roberts, MN., Wallace, MA., Tomilov, AA., et al. A Ketogenic Diet Extends Longevity and Healthspan in Adult Mice. Cell Metabolism. 2017; 26:539–546
- Volek, JS., Freidenreich, DJ., Saenz, C., et al. Metabolic characteristics of keto-adapted ultra-endurance runners. Metabolism Clinical and Experimental. 2016;65:100-110
- Drenick, EJ., Alvarez, LC., Tamasi, GC., et al. Resistance to Symptomatic Insulin Reactions after Fasting. The Journal of Clinical Investigation. 1972;51:2757-2762
- Cahill GF Jr, Aoki TT. Alternate fuel utilization by the brain. In: Cerebral Metabolism and Neural Function. Janet V. Passonneau et al, Eds. Pages 234-242. Williams & Wilkins, Baltimore 1980.
- Cunnane, SC., Courchesne-Loyer, A., Vandenbeghe, C., et al. Can Ketones Help Rescue Brain Fuel Supply in Later Life? Implications for Cognitive Health during Aging and the Treatment of Alzheimer’s Disease. Frontiers in Molecular Neuroscience. 2016;9(53)
- Phinney, SD., Horton, ES., Sims, EAH., et al. Capacity for Moderate Exercise in Obese Subjects after Adaptation to a Hypocaloric, Ketogenic Diet. The Journal of Clinical Investigation. 1980; 66(5):1152-1161
- Phinney SD, Bistrian BR, Kosinski E, Chan DP, Hoffer LJ, Rolla A, Schachtel B, Blackburn GL. Normal cardiac rhythm during hypocaloric diets of varying carbohydrate content. Arch Intern Med. 1983 Dec;143(12):2258-61.
- Reichard Jr., GA., Haff, AC., Skutches, CL., et al. Plasma Acetone Metabolism in the Fasting Human. J Clin Invest. 1978; 63:619-626
- Kiens, B., Essen-Gustavsson, B., Christensen, NJ., et al. Skeletal muscle substrate utilization during submaximal exercise in man: effect of endurance training. The Journal of Physiology. 1993; 49:459-478
- Bergstrom, J., Hultman, E., Jorfeldt, L., et al. Effect of nicotinic acid on physical working capacity and on metabolism of muscle glycogen in man. Journal of Applied Physiology. 1968;26(6)
- Venables MC, Achten J, Jeukendrup AE. Determinants of fat oxidation during exercise in healthy men and women: a cross-sectional study. J Appl Physiol 2005;98(1):160–7
- McKenzie E, Holbrook, T., Williamson, K., et al. Recovery of muscle glycogen concentrations in sled dogs during prolonged exercise. Med Sci Sports Exerc. 2005;37(8):1307-12
- McKenzie EC, Hinchcliff, KW., Valberg, SJ., et al. Assessment of alterations in triglyceride and glycogen concentrations in muscle tissue of Alaskan sled dogs during repetitive prolonged exercise. Am J Vet Res. 2008:69(8):1097-103
- Van Italie TB. Liquid Protein Mayhem. JAMA. 1978;240(2):144-145
- Felig P. Four Questions about Protein Diets. N Engl J Med 1978; 298:1025-1026
- Phinney, SD., Tang, AB., Waggoner, CR., et al. The transient hypercholesterolemia of major weight loss. The American Journal of Clinical Nutrition. 1991; 53(6):1404-1410
- DeHaven, J., Sherwin, R., Hendler, R., et al. Nitrogen and sodium balance and sympathetic-nervous-system activity in obese subjects treated with a low-calorie protein or mixed diet. New Engl J Med. 1980; 302;477-482
- Seligman MJ, et al. Improvement in Patient-Reported Sleep in Type 2 Diabetes and Prediabetes Participants Receiving a Continuous Care Intervention with Nutritional Ketosis. Sleep Med. 2019;55:92-99
- Fried PI, McClean PA, Phillipson EA, Zamel N, Murray FT, Marliss EB. Effect of ketosis on respiratory sensitivity to carbon dioxide in obesity. N Engl J Med. 1976 May 13;294(20):1081-6.